One of the draws of learning about what we eat is coming to understand the origin of foodstuff. I had no idea that gelatin came from animal sources. It made me realize how hard it is to adopt a vegan lifestyle. I truly respect people that do because it takes an enormous amount of effort and awareness to know where products come from and even more discipline to not be a consumer.
That said, I am once again blown away at the ingenuity of the human species. It is remarkable the stuff that people eat and how resourcefulness (i.e. using every bit of an animal/plant) has transformed food. The natural world provides us with so many delicious fruits and vegetables, it is interesting that our palates have craved for more and more diverse ways of consuming them.
On a side note, as I read about pectin and gel-formation, I could not help to reference in my brain that scene from Little Women when Meg is distressed and at her worst presentation when her husband comes home:
John: "My dearest girl, what is the matter?"
Meg: "Oh, John, I am so tired and hot and cross and worried! I've been at it till I'm all worn out. Do come and help me or I shall die!"
John: "What worries you dear? Has anything dreadful happened?"
Meg: "Yes,"
John: "Tell me quick, then. Don't cry. I can bear anything better than that. Out with it, love."
Meg: "The...The jelly won't jell and I don't know what to do!"
(text adapted from Little Women by Louisa M. Alcott, 1869.)
It made me laugh. Poor Meg did not know about pectin, sugar, and acid...
Monday, April 30, 2012
Berry Jam
As noted on Kitchen Chemistry by Dr. Patti Christie
Ingredients:
Method:
Strawberry or other Berry Jam
From Certo package insert
Ingredients:
- 4 cups crushed fruit (this is about 2 quarts of fresh fruit or 2.5 pounds of frozen, thawed fruit)
- 7 cup white sugar
- 1 pouch of liquid Certo
Method:
- Start the dishwasher with the jam jars. The best jars are the Mason Jars with the lid, glass jar and screw bands. To achieve the best seal, it is best to put the jam into a hot glass jar, so time the dishwasher accordingly.
- Prepare fruit. For berries, crush the fruit with a potato masher to the desired chunkiness.
- Measure the exact amount of prepared fruit into a 6 or 8 quart saucepan. It is best to use a pot in which the fruit does not go above about one quarter of the depth to prevent the jam from boiling over
- Stir sugar into fruit
- Bring mixture to full rolling boil ( a boil that does not stop bubbling when stirred) on high heat stirring constantly
- Stir in Certo quickly. Return to full rolling boil and boil exactly 1 minute stirring constantly. Remove from heat. Skim off any foam with a spoon.
- Using a 2 cup measuring cup, ladle quickly into prepared jars, filling to within 1/8 inch of tops. Wipe jar rims and threads. Cover with flat lids, then screw bands tightly.
- Invert jars for 5 minutes, then turn upright.
- After jars cool, check seals by pressing middle of lid with finger. If lid springs back, lid is not sealed and refrigeration is necessary.
- Let stand at room temperature 24 hours. Store unopened jams in a cool dark place up to 1 year. Refrigerate opened jams up to 3 weeks.
- Yield is about 8 cups of jam
Sunday, April 29, 2012
Tips in making a fruit preserve
Cook the fruit to extract the pectin. This preliminary cooking should be brief and as gentle as possible since heat and acid eventually break pectin chains. If a clear jelly is desired, the cooked fruit is strained to remove solid particles and cell debris.
Add sugar and supplemental pectin if needed. Bring the mixture to a rapid boil to concentrate the ingredients. Continue to boil until the mix reaches a temperature of 217-221 degrees F/103 - 105 degrees C at sea level. If not at sea level, adjust the temperature by lowering 2 degrees F/1 degree C per every 1000 ft/305 m elevation. At the noted temperature, the sugar concentration reaches around 65%.
Use a wide pot with a large surface area for evaporation and more gentle cooking.
Add acid in the late stages of cooking to avoid breaking the pectin molecules.
Test the readiness of the mix by placing a drop on a cold spoon and see whether it gels.
Pour into sterilized jars. The mix sets as it cools below 180 degrees F/80 degrees C. It sets most rapidly at warm room temperature (around 86 degrees F/ 30 degrees C), and continous to get firmer with time.
Failure of the mix to set can be attributed to inadequate amounts of good-quality pectin, prolonged cooking that damages the pectin, or inadequate amounts of acid. To remedy, addition of commercial liquid pectin and cream of tartar or lemon juice, and a brief reboiling should work. Too much acid causes weeping of fluid from an overfirm gel.
Uncooked and unsweeted preserves can be made by using concentrated pectin. "Freezer" jams are made by loading crushed fruit with concentrated pectin and sugar and allowed to set for a day. They are preserved in the refrigerator or freezer. Low-calorie and low-sugar jams are made by adding a modified pectin that uses cross-linking calcium to gel instead of sugar. The sugar is replaced by artificial sweeteners.
Add sugar and supplemental pectin if needed. Bring the mixture to a rapid boil to concentrate the ingredients. Continue to boil until the mix reaches a temperature of 217-221 degrees F/103 - 105 degrees C at sea level. If not at sea level, adjust the temperature by lowering 2 degrees F/1 degree C per every 1000 ft/305 m elevation. At the noted temperature, the sugar concentration reaches around 65%.
Use a wide pot with a large surface area for evaporation and more gentle cooking.
Add acid in the late stages of cooking to avoid breaking the pectin molecules.
Test the readiness of the mix by placing a drop on a cold spoon and see whether it gels.
Pour into sterilized jars. The mix sets as it cools below 180 degrees F/80 degrees C. It sets most rapidly at warm room temperature (around 86 degrees F/ 30 degrees C), and continous to get firmer with time.
Failure of the mix to set can be attributed to inadequate amounts of good-quality pectin, prolonged cooking that damages the pectin, or inadequate amounts of acid. To remedy, addition of commercial liquid pectin and cream of tartar or lemon juice, and a brief reboiling should work. Too much acid causes weeping of fluid from an overfirm gel.
Uncooked and unsweeted preserves can be made by using concentrated pectin. "Freezer" jams are made by loading crushed fruit with concentrated pectin and sugar and allowed to set for a day. They are preserved in the refrigerator or freezer. Low-calorie and low-sugar jams are made by adding a modified pectin that uses cross-linking calcium to gel instead of sugar. The sugar is replaced by artificial sweeteners.
High and low pectin fruit
Preserves can be made from all kinds of fruit, though some fruits are low in pectin and have to be mixed with either fruits high in pectin or commercial pectin to get a good gel.
Fruits high in pectin include: quince, apples, citrus fruits, cranberries, currants, gooseberries, plums, and grapes.
Fruits low in pectin include: apricots, most berries, cherries, peaches, pears, and pineapple.
Fruits high in pectin include: quince, apples, citrus fruits, cranberries, currants, gooseberries, plums, and grapes.
Fruits low in pectin include: apricots, most berries, cherries, peaches, pears, and pineapple.
Pectin gels
Pectin is a polysaccharide naturally contained in the cell walls of plants. Coupled with sugar and acid, pectin is what transforms cooked fruits into jams and jellies.
Brief history
The Ancient Greeks discovered that fruit cooked with honey developed a texture unlike any of its counterparts. By the 7th century there were several recipes for making delicate jellies made by boiling the juice of a quince with honey (quince is especially rich in pectin). The introduction of the sugar cane from Asia transformed jelly production. Unlike honey, sugar did not have excess moisture that needed to be evaporated. The Arab world used sugar to make fruit preserves through the Middle Ages, and took them to Europe in the 13th century. Still, sugar jams and jellies did not become common until after the 19th century, when sugar became cheaply available.
Transforming a jelly
Pectin, sugar, and acid are needed to make a fruit preserve, jam, or jelly. When fruit is cut up and heated near the boil, the pectin chains come off of the cell walls and dissolve into the released cell fluids and added water. Pectin molecules have the ability to bond with each other and form a meshwork that gives the jam a gel-like texture. However, pectin cannot accomplish this alone. When in solution, pectin molecules obtain a negative electrical charge that repels them from each other and they are too dilute to form a continuous network. To form the gel, sugar is added to absorb water molecules away from pectin, leaving the long chains exposed for bonding. In addition, boiling concentrates the pectin molecules as excessive water evaporates. Finally, acid provides the needed H+ that neutralize the negative electrical charge of pectin and allows the chains to come in close-enough proximity to bond. The ideal conditions for pectin gelation are: a pectin concentration of 0.5 - 1.0%; a sugar concentration of 60 -65%; and a pH between 2.8 - 3.5, about the acidity of orange juice.
Brief history
The Ancient Greeks discovered that fruit cooked with honey developed a texture unlike any of its counterparts. By the 7th century there were several recipes for making delicate jellies made by boiling the juice of a quince with honey (quince is especially rich in pectin). The introduction of the sugar cane from Asia transformed jelly production. Unlike honey, sugar did not have excess moisture that needed to be evaporated. The Arab world used sugar to make fruit preserves through the Middle Ages, and took them to Europe in the 13th century. Still, sugar jams and jellies did not become common until after the 19th century, when sugar became cheaply available.
Transforming a jelly
Pectin, sugar, and acid are needed to make a fruit preserve, jam, or jelly. When fruit is cut up and heated near the boil, the pectin chains come off of the cell walls and dissolve into the released cell fluids and added water. Pectin molecules have the ability to bond with each other and form a meshwork that gives the jam a gel-like texture. However, pectin cannot accomplish this alone. When in solution, pectin molecules obtain a negative electrical charge that repels them from each other and they are too dilute to form a continuous network. To form the gel, sugar is added to absorb water molecules away from pectin, leaving the long chains exposed for bonding. In addition, boiling concentrates the pectin molecules as excessive water evaporates. Finally, acid provides the needed H+ that neutralize the negative electrical charge of pectin and allows the chains to come in close-enough proximity to bond. The ideal conditions for pectin gelation are: a pectin concentration of 0.5 - 1.0%; a sugar concentration of 60 -65%; and a pH between 2.8 - 3.5, about the acidity of orange juice.
Saturday, April 28, 2012
Types of starch
Grain starches have some common characteristics:
- granules are medium sized
- contain significant amounts of lipids and protein
- have increased structureal stability and thus require higher temperatures to gelate
- have distinct "cereal" flavor
- contain a high proportion of moderately long amylose, so they thicken and congeal quickly
- Wheat flour is only 75% starch, which makes it a less efficient thickener than cornstarch or potatoe starch. It adds a distinct wheat flavor to the sauce. Common rule of thumb is to add 1.5 times as much flour as starch.
- Cornstarch is practically pure starch. It is an efficient thickener, but it absorbs odors and develops flavors during processing.
- Rice starch have the smallest granule size and produce a fine texture. It is seldom available in Western markets.
- larger granules that retain more water molecules
- cook faster
- release starch at lower temperatures
- contain less amylose, but the amylose chains are four times longer than cereal starches
- readily gelate
- do not require precooking to improve flavor
- Potato starch has very large granules and very long amylose chains. Stringiness and initial graininess in the sauces are notable. However, the granules are fragile and fragment easily. It is unusual in that it contains a large number of phosphate groups that carry a weak electric charge and cause the chains to repel each other. This repulsion keeps starch chains evenly dispersed in a sauce and prevents them from congealing when cool.
- Tapioca is derived from the tropical plant casava (aka manioc). It does not develop any strong aromas and it is prized for its neutral flavor. It is mostly used in puddings.
- Arrowroot starch has smaller granules than potato or tapioca starches. Its gelation temperature is higher, more comparable to that of cornstarch.
Starch
Starch is made up of thousands of glucose molecules linked up together. There are two structures of starch molecules: amylose and amylopectin. Amylose molecules are long and straight; amylopectin molecules are short and branched. Of the two, amylose is a more effective thickener than amylopectin as its long chains tangle with each other more readily and slow the motion of other molecules in the surrounding fluid.
When starch is mixed into cold water, its granules only absorb a limited amount of water and sink. Nothing happens. When starch is mixed into hot water, however, the granules absorb large amounts of water and swell up. As they do so, weak regions of the granules become disrupted; stronger regions lose their organized structure and become water-containing meshworks of long molecules. In other words, the granules become individual gels. A cloudy suspension of granules becomes more translucent as individual starch molecules become less packed and no longer deflect as many rays of light.
The temperature at which starch begins to behave in this manner is called the gelation range, usually around 120-140 degrees F/50-60 degrees C.
Thickening of a sauce/dish with starch occurs as the granules become so saturated with water that they begin to leak amylose and amylopectin molecules into the surrounding liquid. The long amylose molecules form a fishnet of sorts that entraps pockets of water and blocks the movement of the swollen starch granules.
After reaching its thickest consistency, the starch-water mixture begins to thin out again. As more amylose leaks into the water, the starch granules break or otherwise become smaller. Heating close to boiling point, vigorous stirring, continued heating long after thickening, and addition of an acid accelerate the thinning process.
The cooling that follows allows amylose molecules to form stable bonds and water molecules settle in the pockets between the starch chains. As a result, the sauce/liquid gets thicker. If the temperature drops low enough, the starch particles begin to congeal. It is important to judge the consistency of a sauce/dish at serving temperatures, not at cooking temperatures. The best way to predict the final texture of a sauce is to pour a spoonful into a cool dish and sample it.
When starch is mixed into cold water, its granules only absorb a limited amount of water and sink. Nothing happens. When starch is mixed into hot water, however, the granules absorb large amounts of water and swell up. As they do so, weak regions of the granules become disrupted; stronger regions lose their organized structure and become water-containing meshworks of long molecules. In other words, the granules become individual gels. A cloudy suspension of granules becomes more translucent as individual starch molecules become less packed and no longer deflect as many rays of light.
The temperature at which starch begins to behave in this manner is called the gelation range, usually around 120-140 degrees F/50-60 degrees C.
Thickening of a sauce/dish with starch occurs as the granules become so saturated with water that they begin to leak amylose and amylopectin molecules into the surrounding liquid. The long amylose molecules form a fishnet of sorts that entraps pockets of water and blocks the movement of the swollen starch granules.
After reaching its thickest consistency, the starch-water mixture begins to thin out again. As more amylose leaks into the water, the starch granules break or otherwise become smaller. Heating close to boiling point, vigorous stirring, continued heating long after thickening, and addition of an acid accelerate the thinning process.
The cooling that follows allows amylose molecules to form stable bonds and water molecules settle in the pockets between the starch chains. As a result, the sauce/liquid gets thicker. If the temperature drops low enough, the starch particles begin to congeal. It is important to judge the consistency of a sauce/dish at serving temperatures, not at cooking temperatures. The best way to predict the final texture of a sauce is to pour a spoonful into a cool dish and sample it.
Other gelling agents
- Carrageenan is a high-molecular weight polysaccharide obtained from red algae and has long been used in China and Ireland. It is a common food additive used as a stabilizer or emulsifier.
- Alginates come from brown seaweeds. They only form gels in the presence of calcium. They are the preferred emulsifiers for ice cream and other dairy products.
- Gellan is an industrial discovery. It is a polysaccharide produced as a fermentation product of the bacteria Sphingomonas elodea. Gellan forms a gel in the presence of salts and/or acids. Gellan is extremely versatile as it forms gels in textures ranging from hard and brittle to fluid. It is used in cosmetics and air-freshners as well as a common additive in food.
From pudding to Petri Dish
In the late 19th century, Lina Hesse, the American wife of a German scientist, recalled the advise of friends who had lived in Asia and made agar jellies and puddings that stayed solid despite the summer heat. Her husband relayed the message to his boss, microbiologist Robert Koch, who then used agar to isolate the causative agent of tuberculosis, Mycobacterium tuberculosis.
Agar gels are used as an invaluable tool in the microbiology laboratory. Scientists make agars containing various nutrients that help grow and differentiate bacteria. Agar is helpful to microbiologists because
Agar gels are used as an invaluable tool in the microbiology laboratory. Scientists make agars containing various nutrients that help grow and differentiate bacteria. Agar is helpful to microbiologists because
- very few bacteria can digest the agar carbohydrates, so agar gels remain intact and bacteria colonies separate. Bacteria readily digest the proteins in gelatin and many liquify the gel.
- Agar gels remain solid at the ideal growth temperature for most bacteria, around 100 degrees F/38 degrees C. Gelatin melts at this temperature.
Agar
Agar is a mixture of several different carbohydrates and other materials extracted from red algae. Today it is manufactured primarily by boiling the seaweeds, filtering the liquid, and freeze-drying it in strands. Solid agar pieces can be consumed raw in salads; it can be used in many sauces as a thickening agent; or used to gel flavorful mixtures of fruit juices and sugar, stews, meats, and vegetables. Agar is consumed as a jellied sweet in Japan.
Agar forms gels at much lower concentrations than gelatin. Where commercial gelatin concentration is usually >3%, agar concentrations work well under 1% by weight. The jelly is somewhat opaque, as opposed to the clear gelatin, and it has a more crumbly texture. Formed agar melts at 185 degrees F/85 degrees C, therefore it does not have the "melt-in-your-mouth" quality of gelatin. Agar gels must be chewed. The higher melting point is often desirable for cool treats that do not melt in the summer heat as well as hot dishes.
Agar forms gels at much lower concentrations than gelatin. Where commercial gelatin concentration is usually >3%, agar concentrations work well under 1% by weight. The jelly is somewhat opaque, as opposed to the clear gelatin, and it has a more crumbly texture. Formed agar melts at 185 degrees F/85 degrees C, therefore it does not have the "melt-in-your-mouth" quality of gelatin. Agar gels must be chewed. The higher melting point is often desirable for cool treats that do not melt in the summer heat as well as hot dishes.
From liquid to solid
When a gelatin solution is hot, the proteins molecules are in constant, forceful movement. As the solution cools, the molecules move more gently. The proteins form regions with helical association, i.e. they coil. A meshwork of gelatin molecules begins to form as these regions bind and align themselves. Liquid becomes trapped in the interstices of the meshwork, preventing a noticeable flow. The liquid becomes a solid gel.
Friday, April 27, 2012
Gelatin
Gelatin is a pure protein derivative that contains no fat, carbohydrates, or cholesterol and is free of all preservatives. It is most widely known for its setting qualities as a thickening and emulsifying agent in culinary uses. However, gelatin is also useful in other food processing, pharmaceuticals, photography, paper production, and other fields.
Gelatin is produced from the partial hydrolysis of collagen found in mammals. It is produced from the connective tissues, bones, and skins of animals, most commonly cows and pigs. The animal tissues undergo a process which includes cleaning, roasting, treating with acid/alkali, and boiling before gelatin is ready to be extracted. Solid gelatin is separated from its liquid components and pressed into sheets. Depending on its final purpose, it is pulverized and mixed with flavorings, colorings, sweeteners, and other additives.
Here is the chemical structure of gelatin:
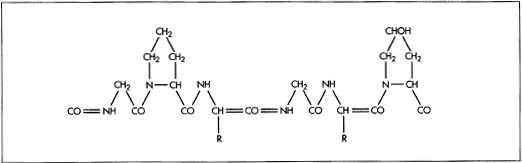
taken from: http://www.madehow.com/Volume-5/Gelatin.html
The chemical structure of gelatin gives it its versatility. It is water-soluble and forms digestible gels and films that are strong and flexible. It is transparent and has no flavor/color.
The exact history of gelatin is not documented. As it is a byproduct of animal parts, it is likely that its discovery dates back hundreds of years. Up to the 19th century, gelatin production was arduous and time consuming. In the 1840s a salesman named Charles B. Knox started packaging sheets of gelatin and selling it door to door. Peter Cooper, who had made a fortune in the manufacture of glue (a similar process to that of gelatin) received the first patent for a gelatin dessert in 1845.
In 1897, Pearl B. Wait, a cough medicine manufacturer, developed a fruit-flavored gelatin. His wife named his product Jell-O. Wait sold the rights to the process to the Genesee Food Company, for $450. In 1902, after an aggressive advertising campaign in Ladies Home Journal magazine generated enormous interest. Today, 400 million packages of Jello-O are produced each year. Over a million packages are purchased or eaten each day.
Gelatin has also had a long presence in other fields. There is documentation of the use of gelatin in paper making as early as the 14th century. In the 1870s, gelatin became a substitute for wet collodion in photography. It was used to coat dry photographic plates, marking the beginning of modern photographic methods. Today, most shells of pharmaceutical capsules are made of gelatin. Glues, paper, cosmetics, soft drinks, and many other things also contain gelatin.
Jams and Jellies
This week is another quick and easy subject. The topics include:
Gelatin jellies
Carbohydrate jellies
Thickeners
Sugar preserves
Hope you join me!
Gelatin jellies
Carbohydrate jellies
Thickeners
Sugar preserves
Hope you join me!
Monday, April 16, 2012
Thoughts on Meringues
The topics of eggs and foams are two of which I knew very little. I read a lot about eggs that I did not publish on posts due to highly politized views on chickens, living conditions, chickens as biological factories, animal rights, and egg economics. Though fascinating, the material is beyond the scope of this blog. If any of these topics piques your interest, I encourage you to research further.
On the posts published, I found some of the figures outstanding:
In terms of techniques, I haven't had much experience making meringues or mousses, but I recently made some macaroons that are similar. I use a pretty efficient stand up mixer, so I did not have any trouble getting stiff peaks, but I imagine that a lot of the trouble with such treats comes down to inefficient beating. It takes a while. If you attempt it by hand, it takes a long, long time. I read somewhere, "whip until both your arms get tired, then ask your neighbor to whip until her arms get tired too, and then some more." Just don't let it get dry or all your efforts have been in vain.
On the posts published, I found some of the figures outstanding:
- Some factories house up to 1 million egg-laying hens, and handle upwards of a million eggs daily.
- A hen expends 3% of her body weight to produce an egg.
- Hens produce around 250 eggs a year in the US.
- There are close to 300 million egg-laying hens in the US (and about 300 million people!)
In terms of techniques, I haven't had much experience making meringues or mousses, but I recently made some macaroons that are similar. I use a pretty efficient stand up mixer, so I did not have any trouble getting stiff peaks, but I imagine that a lot of the trouble with such treats comes down to inefficient beating. It takes a while. If you attempt it by hand, it takes a long, long time. I read somewhere, "whip until both your arms get tired, then ask your neighbor to whip until her arms get tired too, and then some more." Just don't let it get dry or all your efforts have been in vain.
Mile-High Lemon Meringue Tarts
From Women's Day, February 1, 2001, page 124 as posted by Dr. Patti Christie on: http://ocw.mit.edu/courses/special-programs/sp-287-kitchen-chemistry-spring-2009/readings/MITSP_287s09_read07_meringue.pdf
Ingredients:
Lemon filling
Ingredients:
Lemon filling
- 2/3 cup white sugar
- 2 tbps cornstarch
- 2/3 cup water
- yolks from 2 large eggs (reserve whites for meringue)
- 1/3 cup fresh lemon juice (from 1-2 lemons)
- 1 tbsp grated lemon peel (from 1 lemon)
- 1 tbsp butter
- 1 pkg (4 oz) ready-to-serve graham cracker crusts (6/pkg)
- Egg whites from 4 large eggs
- 1/2 tsp Cider vinegar
- 1/2 tsp vanilla extract
- 1/2 cup sugar
Method:
Lemon filling
- Whisk sugar and cornstarch in the top bowl of a double boiler to mix.
- Whisk in water, egg yolks and lemon juice until smooth.
- Place bowl over double boiler, stirring often with the whisk.
- Boil, stirring constantly, 1 minute or until filling is translucent and thick.
- Remove from heat. Add lemon peel and butter; stir until butter melts.
- Pour 1/2 cup into each cracker crust and place on a rimmed baking sheet
- Heat oven to 350 degrees F.
- Beat egg whites, vinegar and vanilla in a medium metal or copper bowl with a whisk until soft peaks form when whisk is lifted.
- Gradually beat in sugar, 1 tbsp. at a time, increasing whisking speed and beating well after each addition until sugar dissolves.
- Beat 2 minutes longer or until stiff peaks form when beaters are lifted.
- Mound Meringue high on each tart, spread to edge of crust , then swirl with back of a teaspoon.
- Bake 20 minutes or until meringue is browned an instant-read thermometer inserted in center of meringue registers 160 degrees F.
- Cool completely on a wire rack, then refrigerate at least 2 hours or up to 8.
- If you wish to share one, use a small sharp knife dipped in cold water to cut through the meringue smoothly.
A note on cold mousses
An egg foam can also be used as scaffolding for cold mousses and cold soufflés. The foam is stabilized when cold congeals fats and gelatin protein. Chocolate mousse is a prime example. Egg yolks are combined with melted chocolate and finely ground sugar and heated to around 100 degrees F/38 degrees C. The mixture is then combined with 3 or 4 times its volume in stiffly beaten egg whites. The cocoa solids and sugar absorbs much of the moisture from the egg whites and the bubble walls are reinforced. The mousse is spooned into dishes and refrigerated for several hours. As the mousse cools down, the cocoa butter congeals into a rigid solid.
Troubleshooting meringues
Meringues have a reputation for being troublesome. Keep in mind the following things:
- Under- or overbeaten foams leak syrup into unsightly beads and puddles.
- Undissolved sugar is undercooked syrup that has come to room temperature. If the sugar has not been completely dissolved, residual crystals attract water and create pockets of concentrated syrup (beads). These meringues have a gritty texture.
- Too high an oven temperature squeezes wter from the coagulating proteins faster than it evaporates, producing syrup beads. It can also cause the foam to rise and crack, and turn its surface yellow.
- Weeping of syrup is caused by either undercooking a foam bottom in a hot oven and cold base, or overcooking the foam bottom in a moderate oven and hot base. To prevent weeping, cover the pie base with a layer of crumbs before adding the meringue topping and include starch or gelatin in the foam to help it retain moisture.
- Humidity is bad for meringues. Their sugary surface absorbs moisture from the air and gets sticky. Transfer dried meringues directly from the oven to an airtight container and serve immediately after removing from container.
Meringues
A meringue is a sweetened egg foam that generally stands on its own. Meringues obtain their stiffness and stability from sugar and heat. They are often baked slowly at low oven temperatures of 200 degrees F/93 degrees C. When browned in a hot oven or under a broiler, the surface gets crisp while the interior remains moist.
Meringues have a lot of sugar. The proportion of sugar to egg white ranges from 1:1 to 2:1. The higher, a 67% sugar content, is typical of jams. At this concentration, sugar reaches its water solubility at room temperature. Granulated sugar does not dissolve completely in a hard meringue. It leaves a gritty texture and weeping syrup drops. Confectioner's sugar or premade syrup are better suited for meringue use.
There are two main categories of meringues: uncooked and cooked.
Uncooked meringues are the simplest and most common. They vary in purpose and firmness based on the timing of the cook's addition of sugar. The lightest meringues are obtained by folding in the sugar only after the foam is formed. The sugar dissolves into the bubble walls and adds bulk and cohesiveness. These meringues are suitable for a spread pie topping or folding into a mousse or chiffon mix. If the sugar is beaten into the foam as it forms, it noticeably tightens the foam texture. These meringues get stiffer and can be shaped. Some cooks reverse the technique by beating the eggs gradually into the sugar. This process takes longer but a cook can form a foam in autopilot as it demans little attention. The resulting meringues are denser and less brittle. Therefore, the earlier the sugar is added, the firmer and finer the meringue.
Cooked meringues are more complex and are generally more dense. Heat incorporates the sugar better in the meringue and stabilizes the protein network into a solid structure. There are two basic cooked meringues:
Meringues have a lot of sugar. The proportion of sugar to egg white ranges from 1:1 to 2:1. The higher, a 67% sugar content, is typical of jams. At this concentration, sugar reaches its water solubility at room temperature. Granulated sugar does not dissolve completely in a hard meringue. It leaves a gritty texture and weeping syrup drops. Confectioner's sugar or premade syrup are better suited for meringue use.
There are two main categories of meringues: uncooked and cooked.
Uncooked meringues are the simplest and most common. They vary in purpose and firmness based on the timing of the cook's addition of sugar. The lightest meringues are obtained by folding in the sugar only after the foam is formed. The sugar dissolves into the bubble walls and adds bulk and cohesiveness. These meringues are suitable for a spread pie topping or folding into a mousse or chiffon mix. If the sugar is beaten into the foam as it forms, it noticeably tightens the foam texture. These meringues get stiffer and can be shaped. Some cooks reverse the technique by beating the eggs gradually into the sugar. This process takes longer but a cook can form a foam in autopilot as it demans little attention. The resulting meringues are denser and less brittle. Therefore, the earlier the sugar is added, the firmer and finer the meringue.
Cooked meringues are more complex and are generally more dense. Heat incorporates the sugar better in the meringue and stabilizes the protein network into a solid structure. There are two basic cooked meringues:
- The Italian meringue is a syrup-cooked meringue. Sugar is made into a syrup first by heating sugar and water to 240 degrees F/115 degrees C. The whites are whipped to stiff peaks and then the syrup is streamed into the foam and beaten. The result is a fluffy, fine-textured, stiff foam. It can be used to decorate pastries or blend into batters and creams. Note: The heat from the hot syrup is not enough to kill salmonella as much of its heat gets lost to the bowl, whisk, and air.
- The Swiss meringue (similar to the French meringue cuite) is prepared by heating eggs, acid, and sugar in a hot bath and then beaten into a stiff foam. This preparation pausterizes the egg whites before forming the foam. This meringue can be refrigerated for several days and is usually pipped into decorative shapes.
Sunday, April 15, 2012
Foam stages
At the glossy "soft peak" stage, foam retains some shape but droops. The foam does not cling to the bowl. Liquid is still likely to drain to the bottom of the bowl.
At the glossy "stiff peak" stage", foam retains a well-defined edge and clings to the bowl. The foam approaches 90% air and the egg liquid is spread thin. There is just enough lubrication to make the foam creamy and mix easily with other ingredients. This is the optimum stage for mousses, soufflés, sponge cakes, and similar dishes.
If the foam is beaten past the glossy stiff peak stage, it enters into the slip-and-streak stage. The appearance is dull and dry. The foam has a crumbly consistency and begins to leak liquid. The proteins in this stage are bonding so closely that they squeeze out the liquid between them. It no longer clings to the bowl. Pastry chefs use this stage for meringues and cookies, and then stop the overcoagulation by adding sugar which separates the proteins again and absorbs the water. Past this stage, the foam loses volume and gets grainy.
At the glossy "stiff peak" stage", foam retains a well-defined edge and clings to the bowl. The foam approaches 90% air and the egg liquid is spread thin. There is just enough lubrication to make the foam creamy and mix easily with other ingredients. This is the optimum stage for mousses, soufflés, sponge cakes, and similar dishes.
If the foam is beaten past the glossy stiff peak stage, it enters into the slip-and-streak stage. The appearance is dull and dry. The foam has a crumbly consistency and begins to leak liquid. The proteins in this stage are bonding so closely that they squeeze out the liquid between them. It no longer clings to the bowl. Pastry chefs use this stage for meringues and cookies, and then stop the overcoagulation by adding sugar which separates the proteins again and absorbs the water. Past this stage, the foam loses volume and gets grainy.
Egg, bowl, and whisk
Just about any egg, bowl, and whisk can provide a good foam. Here are some tips to consider:
- Older eggs at room temperature have thinner whites and foam more quickly, but by the same token a thin egg white is more likely to drain and older yolks rupture more quickly, often leaving traces of yolk in the white.
- Fresh eggs take longer to beat but make a more stable foam.
- Cold yolks are easier to separate from whites. The whipping process warms the whites anyway.
- Egg foams can also be whipped from dried egg whites.
- Use a bowl large enough to accomodate an eightfold expansion of volume.
- If beating by hand, a large "balloon whisk" aerates a greater volume of whites at a time.
- When using a mixer, choose a beater that both spins and traces a curlicue path for a more even foam. Other mixers and beaters work, but less efficient beaters produce a denser texture.
Effects of other ingredients
- Salt weakens structure as its ions Na+ Cl- compete for bonding sites on the unfolded proteins. It's best to add salt to the other components of a dish rather than the foam itself.
- Sugar both hinders and helps foam making. When added early, it delays foaming and reduces the ultimate volume and lightness. At this point, the sugar interferes with the unfolding and bonding of the proteins. The syrup-egg mixture is also heavier and harder to spread into bubbles. It takes twice as long to foam. However, sugar improves foam stability by slowing down drainage from bubble walls. In the oven, dissolved sugar delays moisture loss until after ovalbumin has had time to coagulate and reinforce the foam. Sugar also adds its own reinforcement to the structure in the form of cotton-candy-like strands of dry sugar. Most times, sugar is added to the egg whites after the foam has already begun to form, though sometimes its added at the beginning for a more firm, dense foam.
- Water in small amounts can increase the volume and lightness of foam, but since it thins whites, it is more likely that some liquid drains from the foam.
Protecting the foam
Though proteins are responsible for giving foams structure, they can also destabilize them. If whipped too long, the proteins form tight bonds that squeeze out the water. This causes the foam to lose volume, become grainy, and separate into a dry froth and runny liquid. There are simple ways to prevent this catastrophic collapse:
The presence of copper or silver in foaming egg whites binds to reactive sulfur groups. This renders the proteins unable to form other strong bonds, which prevents proteins from getting too close to each other and collapsing the foam. For this reason, the use of a copper bowl for whipping egg whites has long been recommended. If a copper or silver plated bowl is not available, the cook may add a pinch of powdered copper supplement to obtain the same result.
Bonds with sulfur groups can also be prevented by adding an acid such as 1/8 tsp cream of tartar or 1/2 tsp of lemon juice. The acid boosts the number of available hydrogen (H) ions in the mix, making it harder for the S-H groups to shed their hydrogen and form strong sulfur bonds with anything else.
Other things to keep in check include egg yolks, fats/oils, and detergent. Traces of these three things interfere with protein bonding. Their presence does not prevent a foam from forming, but it takes longer and the quality suffers. Fats and yolks can be added once the foam is formed.
The presence of copper or silver in foaming egg whites binds to reactive sulfur groups. This renders the proteins unable to form other strong bonds, which prevents proteins from getting too close to each other and collapsing the foam. For this reason, the use of a copper bowl for whipping egg whites has long been recommended. If a copper or silver plated bowl is not available, the cook may add a pinch of powdered copper supplement to obtain the same result.
Bonds with sulfur groups can also be prevented by adding an acid such as 1/8 tsp cream of tartar or 1/2 tsp of lemon juice. The acid boosts the number of available hydrogen (H) ions in the mix, making it harder for the S-H groups to shed their hydrogen and form strong sulfur bonds with anything else.
Other things to keep in check include egg yolks, fats/oils, and detergent. Traces of these three things interfere with protein bonding. Their presence does not prevent a foam from forming, but it takes longer and the quality suffers. Fats and yolks can be added once the foam is formed.
Egg foams
Usually I expand more on the basic topic, but as eggs are one of the most versatile food items, I am going to skip and focus the conversation on information that is relevant to the meringue recipe.
The foaming power of egg whites came around the 17th century. At the time, the fork was a still a novelty. Cooks used twigs, bits of dried fruit, or bundle straw to whip the whites into meringues and soufflés.
Physical agitation usually breaks structure, but in egg whites it actually creates structure. Agitation of the sticky egg white transforms it into white foam, a cohesive structure that holds its own when mixed and cooked. Whipping exerts physical stress that unfolds the compact protein molecules. Air becomes incorporated into the whites. It creates an imbalance of water and air that promotes bonding of globulin proteins and ovotransferrin. The bonded proteins become a solid network that pervades the bubble walls and hold water and air in place. Thus the egg white becomes a semi-solid mass of minute bubbles called a foam.
Left alone the foam eventually collapses. Heat transforms it into a permanent solid. Ovalbumin does not contribute much to the foam network, but heat makes it unfold and coagulate. In a cooked foam, ovalbumin more than doubles the amount of solid protein reinforcement in the bubble walls. As water evaporates, the semiliquid foam turns into a solid.
The foaming power of egg whites came around the 17th century. At the time, the fork was a still a novelty. Cooks used twigs, bits of dried fruit, or bundle straw to whip the whites into meringues and soufflés.
Physical agitation usually breaks structure, but in egg whites it actually creates structure. Agitation of the sticky egg white transforms it into white foam, a cohesive structure that holds its own when mixed and cooked. Whipping exerts physical stress that unfolds the compact protein molecules. Air becomes incorporated into the whites. It creates an imbalance of water and air that promotes bonding of globulin proteins and ovotransferrin. The bonded proteins become a solid network that pervades the bubble walls and hold water and air in place. Thus the egg white becomes a semi-solid mass of minute bubbles called a foam.
Left alone the foam eventually collapses. Heat transforms it into a permanent solid. Ovalbumin does not contribute much to the foam network, but heat makes it unfold and coagulate. In a cooked foam, ovalbumin more than doubles the amount of solid protein reinforcement in the bubble walls. As water evaporates, the semiliquid foam turns into a solid.
Tips on egg handling and storage
- Egg grades are classified by the USDA as an approximation of quality at the time the egg was laid. Only the top two grades, AA and A are seen in stores.
- Eggs have the ability to remain edible for weeks as long as they are kept intact and cool. Egg deterioration begins as soon as the egg is laid. The egg suffers changes in pH from acidic to alkaline. At more alkaline pH, the egg whites clear as proteins repel each other instead of forming light-blocking complexes. The whites become more runny. The yolk becomes weaker due to an influx of water that increases its volume and stretches its membranes.
- One can test egg freshness by placing eggs in a container filled with water. Fresh eggs sink rapidly to the bottom. Older eggs stay afloat longer.
- An egg deteriorates as much in a day at room temperature as in four days under refrigeration.
- It's best to buy eggs out of a cooler, not from an open shelf. Keep cold.
- An airtight container slows moisture loss and absorbion of odors.
- To freeze eggs, remove them from the shell. Freeze whites separate from yolks. It's best to mix yolks with a 1 tsp salt, 1 tbsp of sugar, or 4 tbsp lemon juice per pint to prevent proteins from aggregating and obtaining a pasty consistency once thawed. To freeze whole eggs, half the treatment for yolks.
- Salmonella infection of eggs is low, but still a problem. Salmonella is killed if eggs are cooked at 140 degrees F/60 degrees C for 5 minutes, or at 160 degrees F/70 degrees C for 1 min.
- If eggs are to be consumed or used raw, try pasteurized eggs or freeze dried eggs.
- One can tell the difference between a cooked egg vs a raw egg by giving it a spin on its side. If its smooth, then it's cooked. If it is wobbly, it is raw.
Saturday, April 14, 2012
The white
The white accounts for two thirds of the shelled egg's weight. It is made of 90% water. The rest is made up of proteins, minerals, fatty materials, vitamins, and glucose.
The proteins in the whites play important roles in protecting the embryo. Some proteins block the action of digestive enzymes. Others bind tightly to vitamins and/or iron. One protein inhibits the reproduction of viruses, and another digests the cell walls of bacteria. The egg white is a chemical shielf against infection and predation.
The following proteins are important for the cook:
The proteins in the whites play important roles in protecting the embryo. Some proteins block the action of digestive enzymes. Others bind tightly to vitamins and/or iron. One protein inhibits the reproduction of viruses, and another digests the cell walls of bacteria. The egg white is a chemical shielf against infection and predation.
The following proteins are important for the cook:
- Ovomucin accounts for <2% of albumen but has the most influence on the eggs culinary value. Ovomucin pulls together the proteins into an organized structure. It is what makes the thick egg white thick. It gradually desintegrates, so older eggs have a more runny texture.
- Ovalbumin is the most dominant egg protein. Its role is not entirely understood but it is thought to help inhibit protein-digestive enzymes. It is the only egg protein to have reactive sulfur groups, which strongly contributes to the flavor, texture, and color of cooked eggs. Ovalbumin becomes more heat resistant as the egg ages, so fresher eggs need less cooking than older eggs.
- Ovotransferrin holds tightly to iron. It is the first protein to coagulate when an egg is heated, thus it determines the setting temperature. Whole eggs set at higher temperature than egg whites because ovotransferrin binds to the iron in the yolk and becomes more resistant to coagulation. Ovotransferrin changes color when bound to metals, which is why whites whipped in a copper bowl turn golden and whites supplemented with ground-iron turn pink.
The yolk
The yolk accounts for over a third of the eggs weight and its purpose is almost exclusively nutritive. It carries three-quarters of the calories of the egg and most of the iron, thiamin, and vitamin A. It obtains its color from the plant pigments xanthophylls found in the hen's feed. The yolk also contains amylase, a starch-digesting enzyme.
The yolk consists of spherical compartments and tightly packed. These chambers are filled with water that suspend tiny subspheres that contain water and phosphorus-rich proteins where the iron in an egg is bound. Suspended in the water inside these subspheres are low density lipoproteins that contain aggregates of fat, protein, cholesterol and phospholipids, mainly lecithin.
Cholesterol is an essential component of animal cell membranes, therefore, it is a substantial component in the egg. One large egg contains around 215 mg of cholesterol, while an equivalent portion of meat has about 50 mg. With that said, concerns of high blood cholesterol and increased risk of heart disease have placed dietary restrictions on yolk consumption. However, recent studies suggest that egg consumption has little effect on blood cholesterol which is more susceptible to saturated fats than cholesterol itself. Most of the fat in egg yolks is unsaturated.
Egg yolks are far less allergenic than whites and can be safely consumed by infants.
The yolk consists of spherical compartments and tightly packed. These chambers are filled with water that suspend tiny subspheres that contain water and phosphorus-rich proteins where the iron in an egg is bound. Suspended in the water inside these subspheres are low density lipoproteins that contain aggregates of fat, protein, cholesterol and phospholipids, mainly lecithin.
Cholesterol is an essential component of animal cell membranes, therefore, it is a substantial component in the egg. One large egg contains around 215 mg of cholesterol, while an equivalent portion of meat has about 50 mg. With that said, concerns of high blood cholesterol and increased risk of heart disease have placed dietary restrictions on yolk consumption. However, recent studies suggest that egg consumption has little effect on blood cholesterol which is more susceptible to saturated fats than cholesterol itself. Most of the fat in egg yolks is unsaturated.
Egg yolks are far less allergenic than whites and can be safely consumed by infants.
Egg shape
The following picture details the inner structure of a chicken egg:
taken from: http://food.oregonstate.edu/learn/egg.html
Keeping in mind the picture above, let's explore the structure of the egg.
The egg begins with the germ cell atop the yolk. The germ cell contains the hen's chromosomes. Over time, the germ cell accumulates a white, primordial form of yolk, the white yolk. Close to the time when the hen is preparing to lay the egg, the germ cell accumulates yellow yolk, which is mostly composed of fats and proteins. The color of the yolk reflects the pigments in the hen's diet. The yolk becomes large enough to potentially feed a developing chick for 21 days.
The yolk is released from the hen's ovary into the oviduct, where the germ cell can be fertilized if the hen mates. Most eggs are not fertilized. The egg white forms from proteins secreted here, mainly albumen (albumin proteins particular to eggs). Four alternating thick and thin layers of protein form the egg whites that surround the yolk. The chalazae is a twisted albumen anchor that connects the yolk to the shell. It allows the yolk to rotate while suspended in the middle of the egg.
On the outer side of the white, lies an antimicrobial protein membrane that connects everywhere except for the air pocket that is available for the hatching chick to breathe. The shell consists of calcium carbonate and proteins. Finally, a thin proteinaceous cuticle covers the shell which initially plugs the pores in the shell. The cuticle prevents water loss and blocks bacteria from entering the egg. Gradually, the cuticle fractures for the purpose of allowing oxygen in for the developing chick.
The color of the egg comes along with the cuticle. Egg color is determined by the genetic make up of the hen. If the hen has white plumage, the egg is white. If the hen is pigmented, such as the Rhode Island Reds, the eggs are brown. There is neither a nutritional nor a taste difference between brown eggs and white eggs.
Note: This post reminded me of one of my favorite essays by E.B. White, Riposte, where he discusses the preferences of the brown egg versus the white egg. I highly recommend it for an entertaining, and logical view of color.
Friday, April 13, 2012
Evolution and history of eggs
The egg is one of two reproductive cells (the other being the sperm cell), which when fertilized accomodates two gene sets, divides, and differentiates into the embryonic organism. It provides food for the inital stages of growth. Eggs are nutritious because they are designed to be food; they are designed to support new creatures until they are able to fend for themselves.
History dates back to the development of sexual reproduction in multiple-cell organisms. Around 300 million years ago, land dwelling reptiles developed self-contained eggs with shells that prevented fatal moisture loss and provided enough nutrients to support embryonic development into a fully formed animal. About 100 million years later, birds came along. Bird eggs developed a mineralized shell and antimicrobial defenses. These refinements made bird eggs a perfect human food. The egg contains a sizeable and balanced portion of animal nutrients, it is pre-packaged, and it stores well with little or no effort.
The genus Gallus, to which the chicken belongs, came along around 8 million years ago; but the chicken did not become domesticated until around 7500 BCE. From then, the eggs of different fowl made their way through many cultures and peoples. Chickens became prized because of their egg-laying behavior. Some fowl will only lay a certain number of eggs at a time, no matter what happens to the eggs. Others, including chickens, will lay eggs until they accumulate a certain number in their nest. If one of the eggs is taken away, the chicken will lay another to replace it and may do so indefinitely. Humans became the unrelenting predator who bound chickens to lay eggs day after day.
Bird eggs have been cooked probably since the discovery of fire. Salting and pickling eggs were performed by the Ancient. By the time of the Romans, many different recipes and uses were found for eggs. By medieval times, the French had mastered omelets. Yolk-based sauces and egg-white foams developed after the Renaissance.
Between 1850 and 1900, the chicken underwent an evolutionary change. The West was introduced to the Cochin, a Chinese breed of chicken, and a "hen fever" exploded. Hundreds of new breeds were developed. Breeds became prized for their meat or their egg-laying abilities. Today, most of the genetic diversity generated has disappeared. In the US, most chickens today are the product of four purebred grandparents.
The 20th century brought changes in industrialization and mass production. Today, most of the eggs come from egg factories, some of which house up to 1 million egg-laying hens. Most hens live in a cage-system which standarizes diet and social development; and streamlines egg collection, washing, and packaging. Hens in the US lay an average of 250 eggs a year, and suffer less than 5% mortality rate as opposed to free-range hens in the previous century, which laid around 100 eggs a year and suffered 40% mortality rate. The differences are attributed to improved housing (less exposure to the elements, disease, pecking orders, and predators), and more equitatable feeding. Furthermore, mass production exploded as refrigeration made fresh eggs readily and cheaply available. In many areas, eggs are available to the consumer within a day of it being laid by the hen.
Concerns have been raised about making chickens into biological machines. "Free-range," "organically-fed," and other specialty eggs have become available. While there is a benefit to their availability, people pay a hefty price for their concern, as much as 3x more. Even so, there are no clear guidelines as to what those labels mean. "Free range" in some cases only means a larger cage. Switzerland is the only country that requires that all hens have free access to the outdoors.
History dates back to the development of sexual reproduction in multiple-cell organisms. Around 300 million years ago, land dwelling reptiles developed self-contained eggs with shells that prevented fatal moisture loss and provided enough nutrients to support embryonic development into a fully formed animal. About 100 million years later, birds came along. Bird eggs developed a mineralized shell and antimicrobial defenses. These refinements made bird eggs a perfect human food. The egg contains a sizeable and balanced portion of animal nutrients, it is pre-packaged, and it stores well with little or no effort.
The genus Gallus, to which the chicken belongs, came along around 8 million years ago; but the chicken did not become domesticated until around 7500 BCE. From then, the eggs of different fowl made their way through many cultures and peoples. Chickens became prized because of their egg-laying behavior. Some fowl will only lay a certain number of eggs at a time, no matter what happens to the eggs. Others, including chickens, will lay eggs until they accumulate a certain number in their nest. If one of the eggs is taken away, the chicken will lay another to replace it and may do so indefinitely. Humans became the unrelenting predator who bound chickens to lay eggs day after day.
Bird eggs have been cooked probably since the discovery of fire. Salting and pickling eggs were performed by the Ancient. By the time of the Romans, many different recipes and uses were found for eggs. By medieval times, the French had mastered omelets. Yolk-based sauces and egg-white foams developed after the Renaissance.
Between 1850 and 1900, the chicken underwent an evolutionary change. The West was introduced to the Cochin, a Chinese breed of chicken, and a "hen fever" exploded. Hundreds of new breeds were developed. Breeds became prized for their meat or their egg-laying abilities. Today, most of the genetic diversity generated has disappeared. In the US, most chickens today are the product of four purebred grandparents.
The 20th century brought changes in industrialization and mass production. Today, most of the eggs come from egg factories, some of which house up to 1 million egg-laying hens. Most hens live in a cage-system which standarizes diet and social development; and streamlines egg collection, washing, and packaging. Hens in the US lay an average of 250 eggs a year, and suffer less than 5% mortality rate as opposed to free-range hens in the previous century, which laid around 100 eggs a year and suffered 40% mortality rate. The differences are attributed to improved housing (less exposure to the elements, disease, pecking orders, and predators), and more equitatable feeding. Furthermore, mass production exploded as refrigeration made fresh eggs readily and cheaply available. In many areas, eggs are available to the consumer within a day of it being laid by the hen.
Concerns have been raised about making chickens into biological machines. "Free-range," "organically-fed," and other specialty eggs have become available. While there is a benefit to their availability, people pay a hefty price for their concern, as much as 3x more. Even so, there are no clear guidelines as to what those labels mean. "Free range" in some cases only means a larger cage. Switzerland is the only country that requires that all hens have free access to the outdoors.
Lesson 6: Meringues
This week discussion revolves around the wonderful egg, with an emphasis on the whipping quality of egg whites. Topics include:
An introduction to eggs
Egg foams
Beating techniques
The culminating recipe is a lemon meringue tart. Hope you join me!
An introduction to eggs
Egg foams
Beating techniques
The culminating recipe is a lemon meringue tart. Hope you join me!
Tuesday, April 3, 2012
Thoughts on Challah bread
Bread, "the staff of life." I was amazed at the historical influence that bread has had on language and culture. If one was to study the history of bread more in-depth, one would truly find the history of the world. While this blog has been about ingredients and mechanics of bread making, I could not help turn my thoughts towards the more philosophical questions of bread.
One often hears people comment on the change of pace in modern life. Often times the reference suggests the underlying speed and ease with which we get things. Our physical needs are met with just one trip to the grocery store. We exchange dollars for fruits, vegetables, bread, even sweets. No more preparing, planting, sorting, waiting, baking, etc. It's funny how the thing that we miss, the thing that the "good'ol days" were full of, is work. Yet, we feel more overworked than ever. No time to tend a garden, or make bread at home. Honestly, few days seem to have enough time to make a 30-minute meal. The loss of our time to me, seems to be the loss of appreciation for the things that make us happy. Bread is a prime example.
Consider the time it took to make a loaf of bread 300 years ago. The wheat had to be planted, harvested, milled, turned into flour. The water had to be carried. Other ingredients were worked for, traded, who knows. At last, the lady of the house set out to make bread. The dough was made. It sat. A day or two later, the fire was lit, the oven preheated for hours before. The ashes cleaned, the fire adjusted. The dough was kneaded, formed, and set to bake. For how long, I don't know. Finally a loaf came out. The deliciousness of the bread was secondary, I think, to the substenance it provided. I don't doubt it was delicious. But it was appreciated as the reward of long and hard labor.
Manufacturers today produce dough with good aeriation and gluten structure in 4 minutes. Making bread today still takes time, but the time is not spent by the consumer. The question I am left to ponder then is, am I capable of appreciating a loaf of bread the way our ancestors were? In terms of flavor, our society accepts mass-produced bread with no qualms. It is good enough. Artisan bread tastes better, but is the difference enough? Based on the market, most people don't see a good enough difference to pay $4.50 for an artisan loaf versus $1.20 for Wonder bread.
So where does happiness come in? It is my personal philosophy that we can only appreciate the things that we know, and happiness stems from that knowledge. It is not up to me to say whether today's society is any more or less happy than our bread-making ancestors, but I know for a fact that I can't appreciate bread in the way they did. Maybe instant gratification isn't happiness at all. Maybe happiness is solely connected to the intangibles of time, effort, and labor.
One often hears people comment on the change of pace in modern life. Often times the reference suggests the underlying speed and ease with which we get things. Our physical needs are met with just one trip to the grocery store. We exchange dollars for fruits, vegetables, bread, even sweets. No more preparing, planting, sorting, waiting, baking, etc. It's funny how the thing that we miss, the thing that the "good'ol days" were full of, is work. Yet, we feel more overworked than ever. No time to tend a garden, or make bread at home. Honestly, few days seem to have enough time to make a 30-minute meal. The loss of our time to me, seems to be the loss of appreciation for the things that make us happy. Bread is a prime example.
Consider the time it took to make a loaf of bread 300 years ago. The wheat had to be planted, harvested, milled, turned into flour. The water had to be carried. Other ingredients were worked for, traded, who knows. At last, the lady of the house set out to make bread. The dough was made. It sat. A day or two later, the fire was lit, the oven preheated for hours before. The ashes cleaned, the fire adjusted. The dough was kneaded, formed, and set to bake. For how long, I don't know. Finally a loaf came out. The deliciousness of the bread was secondary, I think, to the substenance it provided. I don't doubt it was delicious. But it was appreciated as the reward of long and hard labor.
Manufacturers today produce dough with good aeriation and gluten structure in 4 minutes. Making bread today still takes time, but the time is not spent by the consumer. The question I am left to ponder then is, am I capable of appreciating a loaf of bread the way our ancestors were? In terms of flavor, our society accepts mass-produced bread with no qualms. It is good enough. Artisan bread tastes better, but is the difference enough? Based on the market, most people don't see a good enough difference to pay $4.50 for an artisan loaf versus $1.20 for Wonder bread.
So where does happiness come in? It is my personal philosophy that we can only appreciate the things that we know, and happiness stems from that knowledge. It is not up to me to say whether today's society is any more or less happy than our bread-making ancestors, but I know for a fact that I can't appreciate bread in the way they did. Maybe instant gratification isn't happiness at all. Maybe happiness is solely connected to the intangibles of time, effort, and labor.
Challah (Braided) Bread recipe
As posted by Dr. Patti Christie on http://ocw.mit.edu/courses/special-programs/sp-287-kitchen-chemistry-spring-2009/readings/MITSP_287s09_read05_Challah.pdf
Adapted from the Guggenheim Family, Zurich, Switzerland.
Ingredients:
Adapted from the Guggenheim Family, Zurich, Switzerland.
Ingredients:
- 1 package of dehydrated yeast (2 1/4 tsp).
- 1/2 cup sugar
- 2 cups of water at 115 - 120 degrees F.
- enough flour to make a dough (about 5-6 cups)
- 1 tbsp salt
- 2 tbsp oil
- 1 egg
- cooking spray
- egg yolk
- Mix together water and sugar. Make sure the temperature of the solution is between 110-115 degrees F.
- Sprinkle a package of yeast over the top and let sit for 10 minutes.
- Mix together egg, oil, and salt. Add to yeast mixture.
- Mix in the flour. The total amount of flour will be between 5-6 cups. Add 2 cups of flour and mix. Then add another 2 cups and mix well. Gradually add the rest of the flour to make a dough that is able to come off the sides of the bowl.
- Knead together until the dough is shiny and elastic.
- Spray cooking spray on bowl. Place dough into bowl and flip until covered with cooking spray.
- Cover with a piece of plastic wrap, which has been sprayed with cooking spray and also a towel. Let rise in a warm place (works best if the temperature of the dough is between 85-95 degrees F) until double in bulk. This will take about an hour.
- Carefully punch down and divide the dough into pieces to be braided.
- Braid the bread and cover with the plastic wrap and towel to let rise for about 30 minutes (half the amount of time as the first rising).
- Mix equal parts of egg yolk and water. Brush egg yolk mixture on top of dough.
- Cook at 350 degrees F for 35-45 minutes or until it sounds hollow when tapped.
Staling
Though staling seems to involve the loss of moisture as the bread becomes dry, hard, and crumbly, it is more of an effect of starch retrogradation than net water loss. In 1852, Frenchman Jean Baptiste Boussingault conducted an experiment where bread was hermetically sealed to prevent moisture loss, yet it still became stale. Staling is now understood to be a manifestation of recrystallization and water migration out of the starch granules into the surrounding gluten network. The retrogradation of simple amylsoe molecules leads to the hardening of the bread. Branched amylopectins also retrograde, but do so more slowly. Certain emulsifying agents have been found to retard staling substantially. Manufacturers have added these to mass-produced bread dough for the last 50 years. True buttermilk and eggs have the same effects.
As long as the loaf is not too old, or has been refrigerated, staling can be reversed by reheating the bread above the gelation temperature of starch, 140 degrees F/60 degrees C. Toasting makes the interior of bread soft. A loaf of bread can be refreshed by heating in the oven.
Refrigerating bread can speed up the staling process by as much as 6 days! If bread is to be used in a couple of days, it is best to store at room temperature in a bread box or paper bag to reduce moisture loss. If bread is to be kept longer, it is best to wrap it in plastic or foil, and freeze it. Refrigerate bread only if it is to be toasted or reheated before consumption.
Bread tends to dry out before becoming spoiled. Still, bread that is kept at room temperature in a plastic bag allows moiture from the staling starch granules to collect on bread surfaces. The most commonly found toxic molds in spoiled bread are Aspergillus, Penicillium, Mucor, and Monilia sitophila.
As long as the loaf is not too old, or has been refrigerated, staling can be reversed by reheating the bread above the gelation temperature of starch, 140 degrees F/60 degrees C. Toasting makes the interior of bread soft. A loaf of bread can be refreshed by heating in the oven.
Refrigerating bread can speed up the staling process by as much as 6 days! If bread is to be used in a couple of days, it is best to store at room temperature in a bread box or paper bag to reduce moisture loss. If bread is to be kept longer, it is best to wrap it in plastic or foil, and freeze it. Refrigerate bread only if it is to be toasted or reheated before consumption.
Bread tends to dry out before becoming spoiled. Still, bread that is kept at room temperature in a plastic bag allows moiture from the staling starch granules to collect on bread surfaces. The most commonly found toxic molds in spoiled bread are Aspergillus, Penicillium, Mucor, and Monilia sitophila.
Making bread
There are four main steps in making yeast bread.
Mixing
The first step is to mix the ingredients-flour, water, yeast, and salt-together. During mixing, starch granules absorb water and enzymes digest starch into sugars. The yeast feed on the sugars and produce carbon dioxide and alcohol. Glutenin proteins begin to react into gluten. Mixing can be done by hand, with an electric-mixer, or in a food processor. The later offer the advantage of limited oxygen exposure, which in excess can alter the pigments and flavor of the bread.
Kneading
The second step involves dough development. Through kneading, the dough is stretched, folded over, and compressed over and over. This manipulation strengthens the gluten network. It orients proteins to lay side by side which encourages them to form bonds. Overdevelopment of gluten causes bonds to break and turns the dough sticky and inelastic. Bewrae of overdeveloping the dough when kneading mechanically.
Kneading also aerates the dough. The more air pockets formed, the finer the texture of the final bread. Some breads call for minimal kneading. This results in fewer and larger air cells and their corresponding irregular and coarse texture. The gluten is weak and less developed, but it continues to develop through fermentation and can rise to an airy, tender crumb.
Fermentation (Rising)
The third step is fermentation, where the dough is set aside for yeast cells to produce carbon dioxide. As they do so and the carbon dioxide diffuses into air pockets, the dough rises. Yeast have the highest metabolic activity at 95 degrees F. This produces the greatest amount of carbon dioxide and metabolic by-products, some of which can be sour and unpleasant. For quick rising, it is suggested to keep fermentation at 80 degrees F for a couple of hours. Lower temperatures may extend the fermentation an hour or two, but generally produce more desirable flavors.
The end of fermentation is signaled by the dough's volume and the gluten matrix. Fully fermented dough is about twice its original size and has been stretched to its limit, so a finger impression remains when touched. Fermented doughs feel softer and are easier to handle than freshly kneaded dough.
Fermentation can be retarded by storing dough in the refrigerator. Yeast take 10 times as long to rise bread in cool temperatures. Retarding fermentation not only allows bakers to break up the work of making bread, but it has useful effects too. Long, slow fermentation allows greater flavor development by the yeast and bacteria in the dough. Cold dough handles easier without as much loss of leavening gas. The cycle of cooling and rewarming redistributes gases and promotes the development of a more open and irregular crumb structure.
Baking
The last step is baking. The kind of oven where a bread is baked has an important influence on the qualities of the finished loaf. Traditional bread ovens were made out of clay, stone, or brick. The baker preheated the oven by wood fire to temperatures up to 900 degrees F. The domed roof stored heat and radiated it down onto the loaves. The temperature declines during baking. The dough expands early on. The bread benefits from color and flavor development due to enhanced browning reactions.
Modern metal ovens are not ideal for bread making. The maximum cooking temperature is usually around 500 degrees F. Heat cannot be stored as well within their walls, so modern ovens maintain a heat source of gas or electrical elements. The necessary venting does not allow gas ovens to retain the loaves' steam. Some bakers use ceramic baking stones or ceramic oven inserts that mimic the traditional oven. The oven is preheated to its maximum temperature and provide more intensive even heat during baking.
Steam is important in the early baking stages because it increases the rate of heat transfer from the oven to the dough. As steam condenses onto the dough surface, it forms a thin film of water that temporarily prevents it from drying out into a crust. By doing so, it encourages the initial rapid expansion of the loaf. The hot water film eventually dries into an attractive glossy crust. Professional bakers often inject steam in the first minutes of baking. At home, one can spray water or throw ice cubes into the hot chamber to improve oven spring and crust gloss.
There are three stages of baking plus cooling.
Early baking: Oven Spring refers to the first 6-8 minutes of baking. Heat transfers first from the oven floor to the bottom of the dough, and to the top from the hot air and oven ceiling. Heat moves from the surface through the dough slowly through the gluten matrix; and rapidly through the gas network. Alcohol and water in the dough vaporize. The gas cells expand, and the dough rises. The better leavened the dough, the faster it cooks.
Mid-baking: The dough begins to transform into a sponge when the interior temperature of the dough reaches 155-180 degrees F/68-80 degrees C. At this range, the gluten proteins form strong cross-link bonds, the starch granules gelate, and the amylose molecules leak out. Gas pressure builds and ruptures the walls, turning the closed network of bubbles into an open network of pores similar to a sponge.
Late baking: Starch continues to gelate thoroughly. Continued cooking encourages surface browning reactions that improve color and flavor. Though limited to the crust, these reactions affecte the flavor of the whole loaf because their products diffuse downward. Bread is done when its crust has browned and the inner structure has set. Fully cooked bread feels light and hollow.
Cooling
The temperature varies inside a loaf immediately after being removed from the oven. During cooling, the differences even out. Most moisture loss occurs at this stage as moisture in the interior diffuses outward. Small rolls dry out the most, while large loaves the least. As temperature declines, starch granules become firmer, which later allows even slicing. This firming continues over the next day and starts the process of staling.
Mixing
The first step is to mix the ingredients-flour, water, yeast, and salt-together. During mixing, starch granules absorb water and enzymes digest starch into sugars. The yeast feed on the sugars and produce carbon dioxide and alcohol. Glutenin proteins begin to react into gluten. Mixing can be done by hand, with an electric-mixer, or in a food processor. The later offer the advantage of limited oxygen exposure, which in excess can alter the pigments and flavor of the bread.
Kneading
The second step involves dough development. Through kneading, the dough is stretched, folded over, and compressed over and over. This manipulation strengthens the gluten network. It orients proteins to lay side by side which encourages them to form bonds. Overdevelopment of gluten causes bonds to break and turns the dough sticky and inelastic. Bewrae of overdeveloping the dough when kneading mechanically.
Kneading also aerates the dough. The more air pockets formed, the finer the texture of the final bread. Some breads call for minimal kneading. This results in fewer and larger air cells and their corresponding irregular and coarse texture. The gluten is weak and less developed, but it continues to develop through fermentation and can rise to an airy, tender crumb.
Fermentation (Rising)
The third step is fermentation, where the dough is set aside for yeast cells to produce carbon dioxide. As they do so and the carbon dioxide diffuses into air pockets, the dough rises. Yeast have the highest metabolic activity at 95 degrees F. This produces the greatest amount of carbon dioxide and metabolic by-products, some of which can be sour and unpleasant. For quick rising, it is suggested to keep fermentation at 80 degrees F for a couple of hours. Lower temperatures may extend the fermentation an hour or two, but generally produce more desirable flavors.
The end of fermentation is signaled by the dough's volume and the gluten matrix. Fully fermented dough is about twice its original size and has been stretched to its limit, so a finger impression remains when touched. Fermented doughs feel softer and are easier to handle than freshly kneaded dough.
Fermentation can be retarded by storing dough in the refrigerator. Yeast take 10 times as long to rise bread in cool temperatures. Retarding fermentation not only allows bakers to break up the work of making bread, but it has useful effects too. Long, slow fermentation allows greater flavor development by the yeast and bacteria in the dough. Cold dough handles easier without as much loss of leavening gas. The cycle of cooling and rewarming redistributes gases and promotes the development of a more open and irregular crumb structure.
Baking
The last step is baking. The kind of oven where a bread is baked has an important influence on the qualities of the finished loaf. Traditional bread ovens were made out of clay, stone, or brick. The baker preheated the oven by wood fire to temperatures up to 900 degrees F. The domed roof stored heat and radiated it down onto the loaves. The temperature declines during baking. The dough expands early on. The bread benefits from color and flavor development due to enhanced browning reactions.
Modern metal ovens are not ideal for bread making. The maximum cooking temperature is usually around 500 degrees F. Heat cannot be stored as well within their walls, so modern ovens maintain a heat source of gas or electrical elements. The necessary venting does not allow gas ovens to retain the loaves' steam. Some bakers use ceramic baking stones or ceramic oven inserts that mimic the traditional oven. The oven is preheated to its maximum temperature and provide more intensive even heat during baking.
Steam is important in the early baking stages because it increases the rate of heat transfer from the oven to the dough. As steam condenses onto the dough surface, it forms a thin film of water that temporarily prevents it from drying out into a crust. By doing so, it encourages the initial rapid expansion of the loaf. The hot water film eventually dries into an attractive glossy crust. Professional bakers often inject steam in the first minutes of baking. At home, one can spray water or throw ice cubes into the hot chamber to improve oven spring and crust gloss.
There are three stages of baking plus cooling.
Early baking: Oven Spring refers to the first 6-8 minutes of baking. Heat transfers first from the oven floor to the bottom of the dough, and to the top from the hot air and oven ceiling. Heat moves from the surface through the dough slowly through the gluten matrix; and rapidly through the gas network. Alcohol and water in the dough vaporize. The gas cells expand, and the dough rises. The better leavened the dough, the faster it cooks.
Mid-baking: The dough begins to transform into a sponge when the interior temperature of the dough reaches 155-180 degrees F/68-80 degrees C. At this range, the gluten proteins form strong cross-link bonds, the starch granules gelate, and the amylose molecules leak out. Gas pressure builds and ruptures the walls, turning the closed network of bubbles into an open network of pores similar to a sponge.
Late baking: Starch continues to gelate thoroughly. Continued cooking encourages surface browning reactions that improve color and flavor. Though limited to the crust, these reactions affecte the flavor of the whole loaf because their products diffuse downward. Bread is done when its crust has browned and the inner structure has set. Fully cooked bread feels light and hollow.
Cooling
The temperature varies inside a loaf immediately after being removed from the oven. During cooling, the differences even out. Most moisture loss occurs at this stage as moisture in the interior diffuses outward. Small rolls dry out the most, while large loaves the least. As temperature declines, starch granules become firmer, which later allows even slicing. This firming continues over the next day and starts the process of staling.
Sunday, April 1, 2012
Handling and using yeast
Here are a few tips to keep in mind when handling yeast.
- Active dry can be used at 50% of the weight of fresh yeast. Instant dry can be used at 40% of the weight of fresh yeast.
- Yeast responds to warm water only.
- It is extremely sensitive to cold temperatures, too much air, and too much heat.
- Yeast dies within a couple of hours. Though most of the yeast is dead by the time the bread is placed in the oven to bake, there is still enough active yeast to produce carbon dioxide during the early stages of baking. Bread continues to rise in the early stages of baking, but as it progresses, the high temperature kills off the remainder yeast. Most bread deflates slightly toward the end of baking.
- A few things inhibit the yeast's ability to ferment. Salt, shortening, and animal fats can affect the rising action. Recipes that use yeast as the leavening agent, usually contain small amounts of salt and have some sugar for balance.
- Inactivated or dry yeast may at times be dead before use. To check for vitality, add a package to warm water and watch for gas production or expansion. Only after that, combine yeast with flour. Yeast will not activate if the water is too hot, too cold, or if a liquid other than water is used (i.e. milk).
Baker's yeast
There are several types of Baker's yeast:
Cake Yeast, also known as wet, fresh, or compressed yeast, is characterized by a high moisture content. Cake yeast is processed one step further than cream yeast and is taken directly from the fermentation vat. the yeast cells are alive and produce more leaving gas than other forms. It is very perishable and needs to be refrigerated. Shelf life is short, between 2 and 8 weeks.
Active Dry Yeast is yeast is processed one step further than Cake Yeast. It is taken from the fermentation tank and dried into granules with a protective coating of yeast debris. Due to the low moisture content, the yeast is in a semi-dormant state and is therefore more stable than cake yeast. At the time of usage, the baker needs to reactivate the yeast by soaking them in warm water, 105-110 degrees F/41-43 degrees C, before adding mixing into the dough. The shelf life of an unopened package extends from a few months up to 2 years.
Instant Yeast, also known as "fast-rising" or "fast-acting" yeast, is a "dry" yeast that can shorten the rising time in traditional baking by as much as 50%. This yeast is dried quickly and into porous rods instead of granules. This rod formation allows them to take up water more rapidly. Instant yeast does not need to be prehydrated and usually produces more carbon dioxide gas than active dry yeast The shelf life of an unopened package extends up to 2 years.
Cake Yeast, also known as wet, fresh, or compressed yeast, is characterized by a high moisture content. Cake yeast is processed one step further than cream yeast and is taken directly from the fermentation vat. the yeast cells are alive and produce more leaving gas than other forms. It is very perishable and needs to be refrigerated. Shelf life is short, between 2 and 8 weeks.
Active Dry Yeast is yeast is processed one step further than Cake Yeast. It is taken from the fermentation tank and dried into granules with a protective coating of yeast debris. Due to the low moisture content, the yeast is in a semi-dormant state and is therefore more stable than cake yeast. At the time of usage, the baker needs to reactivate the yeast by soaking them in warm water, 105-110 degrees F/41-43 degrees C, before adding mixing into the dough. The shelf life of an unopened package extends from a few months up to 2 years.
Instant Yeast, also known as "fast-rising" or "fast-acting" yeast, is a "dry" yeast that can shorten the rising time in traditional baking by as much as 50%. This yeast is dried quickly and into porous rods instead of granules. This rod formation allows them to take up water more rapidly. Instant yeast does not need to be prehydrated and usually produces more carbon dioxide gas than active dry yeast The shelf life of an unopened package extends up to 2 years.
Yeast metabolism
Baker's and brewer's yeast is Saccharomyces Cerevisiae, also referred to as the "sugar-eating fungus."
Yeasts feast on glucose and fructose from sugar, and on maltose from the broken-down starch granules in the flour.
Yeast metabolize sugars for energy and produce ethyl alcohol and carbon dioxide following this equation:
C6H12O6 -----> 2(CH3CH2OH) + 2(CO2) + ATP
In making beer and wine, the carbon dioxide escapes the liquid and concentrates the alcohol. In making bread, carbon dioxide and alcohol become trapped in the dough. The flexibility of the dough accomodates the expanding gas by inflating or "rising." The ethyl alcohol, along with other by-products of fermentation, give yeast-leavened breads their typical aroma. At the time of baking, the heat expells both carbon dioxide and alcohol from the dough, leaving a flavorful network of empty air pockets.
A small amount of added table sugar increases yeast activity, whereas a large amount decreases it. Too much sugar dehydrates the yeast. To compensate, bakers of sweet breads add more yeast than ordinary, and allow longer times for the bread to rise.
Yeast are also sensitive to salt, and are greatly affected by temperature. Cells grow and produce gas most rapidly at around 95 degrees F/35 degrees C.
Yeasts feast on glucose and fructose from sugar, and on maltose from the broken-down starch granules in the flour.
Yeast metabolize sugars for energy and produce ethyl alcohol and carbon dioxide following this equation:
C6H12O6 -----> 2(CH3CH2OH) + 2(CO2) + ATP
In making beer and wine, the carbon dioxide escapes the liquid and concentrates the alcohol. In making bread, carbon dioxide and alcohol become trapped in the dough. The flexibility of the dough accomodates the expanding gas by inflating or "rising." The ethyl alcohol, along with other by-products of fermentation, give yeast-leavened breads their typical aroma. At the time of baking, the heat expells both carbon dioxide and alcohol from the dough, leaving a flavorful network of empty air pockets.
A small amount of added table sugar increases yeast activity, whereas a large amount decreases it. Too much sugar dehydrates the yeast. To compensate, bakers of sweet breads add more yeast than ordinary, and allow longer times for the bread to rise.
Yeast are also sensitive to salt, and are greatly affected by temperature. Cells grow and produce gas most rapidly at around 95 degrees F/35 degrees C.
History of yeast
The history of yeast dates further back than written language. Egyptian hieroglyphics, dating back 5,000 years, depic the Ancient using yeast for alcohol fermentation as well as a leavening agent. It is believed that early fermentation occurred naturally through contamination of fruit and flour. The Ancient used yeast even though they did not understand it. The faculties of yeast were often attributed to magic.
Leaven, as it is described in the Bible, was a soft dough-like medium. A small portion was reserved as a starter dough for new bread. By saving "good" batches, man naturally selected the best yeast for his baking/fermentation needs. Yeast is therefore considered the first industrial microorganism.
Yeast was not identified as the living organism responsible for fermentation and leavening until after the invention of the microscope in the 1600s and the work of Louis Pasteur in the 1860s. Isolation of pure cultures gave way to commercial production and manufacturing starting at the turn of the 20th century.
Leaven, as it is described in the Bible, was a soft dough-like medium. A small portion was reserved as a starter dough for new bread. By saving "good" batches, man naturally selected the best yeast for his baking/fermentation needs. Yeast is therefore considered the first industrial microorganism.
Yeast was not identified as the living organism responsible for fermentation and leavening until after the invention of the microscope in the 1600s and the work of Louis Pasteur in the 1860s. Isolation of pure cultures gave way to commercial production and manufacturing starting at the turn of the 20th century.
Kinds of flour
Most flours available are labeled according to purpose and usually do not include information about the type of wheat they contain. Flour compositions tend to be blends and can vary from region to region. For example, "all-purpose" flour in the South and Pacific Northwest tends to have lower protein content than flour in other parts of the US and Canada. For this reason, recipes developed in the South turn differently when prepared in other regions unless care is taken to approximate the original. Still, here are some guidelines:
- Whole wheat flours are high in protein; with a high concentration of that protein steming from the germ and aleurone layer, which do not form gluten well. Therefore, they make flavorful but dense bread.
- Bread flours are high in strong gluten proteins. They give the lightest, highest, chewiest loaf breads.
- Pastry flours contain low levels of weak gluten protein. They make tender baked goods.
- Cake flour is soft, low-protein, finely-milled, and strongly bleached with chlorine dioxide or chlorine gas. This treatment enhances the starch granules' ability to absorb water and swell in high-sugar batters. It also helps fat bind more readily to the starch granules' surface, which helps disperse the fat more evenly. Lastly, chlorination also gives the flour an acidic pH. Packaged cake mixes have a high sugar to flour ratio; sugar can outweigh flour by as much as 40%. This allows cakes to have a distinctively light and moist texture.
- "Self-rising" flours contain baking powder (1 1/2 tsp baking powder per cup) and therfore do not require added leavening.
- "Instant" flours are low protein flours whose starch granules have been precooked and dried. Water is more able to penetrate the granules during cooking, such that they are well suited for tender pastries and last-minute thickening of sauces and gravies.
- For pastry flour, add one part by weight of starch to two parts of all-purpose flour.
- For all purpose flour, add one-quarter part of gluten to two parts pastry flour.
Flour
The qualities of flour are determined by the type of wheat (or grain) used and the method of milling.
Milling is the process by which the wheat kernel is broken down and sifted into small particles. Refined flour is sieved to remove the germ and bran layers from the endosperm of the grain. Bran and germ are rich in nutrients and flavor, but they spoil quickly and interfere with the formation of continuous, strong gluten. In conventional milling, grooved metal rollers shear open the grain, squeeze out the germ, and scrape the endosperm away to be ground, sieved, and reground until it attains a specific particle size. Stone grinding is not as widely used, but it crushes the whole grain more thoroughly before sieving, which allows some of the germ and bran to end up in the refined flour. This makes stone ground flour more nutritious and flavorful, but gives it a shorter shelf life.
Freshly milled flour makes weak gluten and dense loaves. Flour needs to be aged to improve its baking qualities. As it ages, flour becomes exposed to oxygen which enhances its ability to make long gluten chains. Oxygen frees sulfur groups at the end of glutenin proteins that allows them to better react with each other. Manufacturers have supplemented flour with oxidizing agents such as ascorbic acid and azodicarbonamide to speed this process. Traditional air-aging also had the secondary effect of lightening the flour, rendering it whiter as yellow xanthophyll pigments oxidized to colorless compounds. To obtain similar whiteness, bleaching flour with azodicarbonamide and peroxide has become common practice in the United States. However, there have been concerns about this chemical alteration. Hence, bleaching is not allowed in Europe.
Gluten proteins and starch account for 90% of flour weight, but there are two minor components that have important effects, fats and enzymes. Fat accounts for only 1% of flour but it is essential to the development of raised bread. Fats help stabilize the bubble walls, help soften the bread structure, and slow staling. Enzymes break down starch to simple sugars that are more easily digested by yeast. Manufacturers have started to add enzymes extracted and purified from microscopic molds ("fungal amylase") to increase enzymatic activity in a predictable pattern.
Milling is the process by which the wheat kernel is broken down and sifted into small particles. Refined flour is sieved to remove the germ and bran layers from the endosperm of the grain. Bran and germ are rich in nutrients and flavor, but they spoil quickly and interfere with the formation of continuous, strong gluten. In conventional milling, grooved metal rollers shear open the grain, squeeze out the germ, and scrape the endosperm away to be ground, sieved, and reground until it attains a specific particle size. Stone grinding is not as widely used, but it crushes the whole grain more thoroughly before sieving, which allows some of the germ and bran to end up in the refined flour. This makes stone ground flour more nutritious and flavorful, but gives it a shorter shelf life.
Freshly milled flour makes weak gluten and dense loaves. Flour needs to be aged to improve its baking qualities. As it ages, flour becomes exposed to oxygen which enhances its ability to make long gluten chains. Oxygen frees sulfur groups at the end of glutenin proteins that allows them to better react with each other. Manufacturers have supplemented flour with oxidizing agents such as ascorbic acid and azodicarbonamide to speed this process. Traditional air-aging also had the secondary effect of lightening the flour, rendering it whiter as yellow xanthophyll pigments oxidized to colorless compounds. To obtain similar whiteness, bleaching flour with azodicarbonamide and peroxide has become common practice in the United States. However, there have been concerns about this chemical alteration. Hence, bleaching is not allowed in Europe.
Gluten proteins and starch account for 90% of flour weight, but there are two minor components that have important effects, fats and enzymes. Fat accounts for only 1% of flour but it is essential to the development of raised bread. Fats help stabilize the bubble walls, help soften the bread structure, and slow staling. Enzymes break down starch to simple sugars that are more easily digested by yeast. Manufacturers have started to add enzymes extracted and purified from microscopic molds ("fungal amylase") to increase enzymatic activity in a predictable pattern.
Subscribe to:
Posts (Atom)